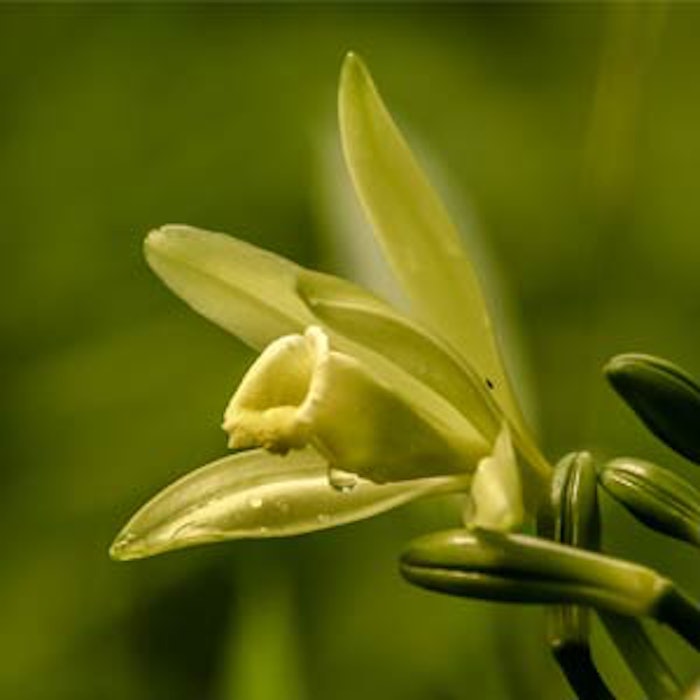
Editor’s note: This is the last article in a series of four describing the chemical characterization of Vanilla pompona. GC-MS and DTD-GC-MS were carried out in Part I to determine the ingredient’s quality as a source for aromatic compounds. Part II provided results and a discussion of the findings. Part III set up the GC-O/MS protocol and provided initial observations. In this final part, results of the GC-O/MS are provided, including a discussion of how the chemical distribution of V. pompona impacts its odor profile.
Results: Identification of Aroma Impact Compounds
As shown in T-1, among the 45 aroma impact compounds identified by their retention index and olfactory quality, 32 were confirmed by their mass spectra.
Despite the capability of AMDIS, additional steps were necessary in the cases of weak mass spectra signals. This occurred for compounds 3, 10, 12 and 15 (see F-1). Here, mass spectra were uncovered by manual deconvolution in AMDIS software using the main fragment ions of the target compounds.
During the identification process, some gaps were observed in retention times (or indices) between mass detection (RI(MS)) and olfactory detection (RI(O)) for later-eluting compounds. To make things even more complicated, the retention time gaps were neither consistent nor exhibited any clear trends. The highest deviation points corresponded to the most crowded region of effluents from the GC column; i.e., during the 20-38 min time range.
The system had been set up for identical retention times for mass spectra and olfactory detection, which was the case for early-eluting compounds. However, it was discovered that high-boiling compounds condensed at the end of the sniff port transfer line, where it was not well-heated. These condensed materials undoubtedly retained the compounds passing through the transfer line, resulting in the unequal delays in retention times obse rved.
The solution to this problem was injecting authentic compounds to determine the retention time gaps whenever there was doubt. As shown in T-1, authentic compounds of numbers 8, 12, 13, 20, 21, 22, 26, 29, 30, 37, 40 and 41 were injected for this purpose. These injected compounds are indicated with the letter I.
Several odor compounds having no presence in the GC-MS data required additional steps for identification. In such cases, the injection of standard compounds was critical to obtain their identities. This was the case for compounds 5, 16, 23 and 27. Volatile aroma compounds were proposed for them based on their distinctive aroma quality and Retention Index (RI(O)), then confirmed via injection of the authentic compounds.
Some odor compounds were not present in the GC-MS data and had no available standard compounds for confirmation; these odorants are listed as tentative, T: 4, 9 and 24 (see T-1). These were identified based on familiar but unique aroma qualities and retention indices; however, they were not confirmed by mass spectra or the injection of authentic compounds.
In the case of compounds 4 and 24, the potency of the odors is an additional factor to consider, in order to narrow down the tentative odorants. For instance, cross-referencing the odor quality and tentative retention index (RI(O)) of compound 4 from olfactory databases led to the belief that unknown compound would be 2,3 octanedione; with dill cooked broccoli buttery odors and an RI(lib) of 1335. However, the unknown compound exhibited higher potency than the typical intensity of the well-known odorant 2,3-octanedione. This higher odor potency or lower detection threshold suggested a more potent and well-known sulfur compound, 2-methyl-3-furanthiol; with cooked meat, fried, potato, roasted meat odors and an RI(lib) of 1319. The odor characteristics of this compound was in good agreement with the odor quality perceived at the sniff port as described in T-1.
Similar criteria were applied to identify compound 24, whose RI and olfactive quality led to its tentative identification as diisopropyldisulfide, with an RI of 1368 on the DB-Wax column. This compound has characteristic medium-low intensity, sulfury tonatily, as obtained from the internal database of previous studies.
Results: Aroma Impact Compounds of V. pompona
This GC-O analysis revealed 45 volatile compounds responsible for the aroma of the V. pompona extract. Among these, thirteen were considered primary aroma-impact compounds, eighteen were identified as secondary aroma-impact compounds and 18 were considered background components.
The chemical structures of the 13 primary aroma-impact compounds of V. pompona are shown in F-2, along with their aroma characteristic, total intensity and detection frequency obtained from the GC-O analysis.
The chemical structures of the 18 secondary aroma-impact compounds of V. pompona are shown in F-3.
The different overall aromas between vanillas of different species are attributed to the various compositions of the aroma impact compounds. In this work, we identified a higher number of impacting odorants (45) in V. pompona. Twenty-six odorants were reported in Vanilla planifolia extract (Perez Silva et al., 2006), and thirty-eight in Vanilla tahitensis extract (Brunschwig et al., 2012). Additional consituents that produce distinctive floral and spice-anise nuances also were found in V. tahitensis (Brunschwig et al., 2012). A significant overlap in aroma-impact compounds between V. pompona and V. tahitensis suggests the two vanilla species might have similar aroma characteristics.
The most important contributors to the overall aroma of V. pompona extract are 1,5Z-octadien-3-one, acetic acid, trans-methyl cinnamate, 2-acetyl-1-pyrroline and 1-octen-3-one because these primary compounds had the highest aroma intensity. These potent odorants also were the primary contributors to the sweet, floral, fruity, nutty, earthy and smoky profile of this extract.
It is worthwhile to note that the sweet and vanillic tonalities of the typical natural vanilla flavor were constant descriptors in most of the primary and secondary aroma impact compounds. This indicated most of the odor compounds relevant to the overall aroma contributed, to some extent, to the strong sweet profile of this vanilla extract.
Sixteen compounds were newly identified by GC-O analysis as aroma impact compounds in V. pompona. These are: ethylpyrazine; 3Z-nonenal; 3-methyl butyric acid; trans-4, 5-epoxy-2E-decenal; 3-octen-2-one; (E,E)-2,6-nonadienal; 10-undecenal; anisyl formate; 3Z-hexenal; diisopropyldisulfide; (E,E)-2,4-nonadienal; 2E-undecenal; phenethanol; acetylpyrrole; trans-geranylacetone; and 5-vinylguaiacol.
The building blocks of the V. pompona aroma, based on the chemical classes of the impact compounds, are presented in F-4. Comparing this chemical distribution with the GC-MS results is interesting in that the aldehydic fraction of the odorants, which represents a small percentage in terms of abundance (5%), contains the largest number of odor-impacting compounds (31%). In contrast, aliphatic alcohols that represent 21% of the total area abundance contributed only to 5% of the aroma of V. pompona extract.
It also is worthwhile to mention that the most abundant compounds according to the GC-MS analysis, i.e., vanillin and anisyl alcohol, were not categorized as primary aroma-impact compounds in the GC-O analysis. This may be explained by the diluting effect caused by the elution in wide peaks that produced long-lasting, strong sweet notes that were difficult to pinpoint at given detection times.
Some compounds, such as ethyl pyrazine and 3-methyl butyric acid, which are present in the extract at low concentrations (Galeas 2015), are important contributors to the overall aroma of V. pompona. The relevance of trace compounds to the aroma of vanillas it is well-known, as has been stressed by Adedeji (1993) and Perez Silva et al. (2006) regarding V. planifolia.
Chemical Distribution of the Aroma-impact Compounds
The odorants identified in this vanilla extract were organized by chemical group (see T-2), including: 14 aldehydes, seven phenolic compounds, five heterocyclic compounds, three esters, four ketones, two acids, two alcohols, two sulfur compounds and six unknowns, each group with distinctive aroma qualities and a sum of total intensities, as described next and in F-4.
Carbonyls, aldehydes (fatty, fruity and floral odors): Odors described as “fatty” and “fruity” are high-quality markers of vanilla aroma and come from unsaturated and saturated aliphatic aldehydes. The most intense contributors to the “fatty” odors in the aroma of V. pompona were 3Z-nonenal and trans-4, 5-epoxy-2E-decenal. It is well-known that aldehydes such as hexanal, octanal and nonanal contribute significantly to the aroma of V. planifolia and V. tahitensis; these also are considered essential to the aroma of V. pompona.
The compounds 10-undecenal, 2E-undecenal and 2E, 4Z-decadienal (see F-3) are responsible for the heliotropin, floral or geranium-like odors in the extract. The compound p-anisaldehyde, which is abundant in the extract, is also an important contributor to the floral fingerprint (see F-2). The sum of the total intensities of the aldehydic fraction of the aroma impact compounds is 3,128; recall from Part III of this series that the sum of all aroma intensity values assigned by the panelists to an odor active compound is referred to as the Total Intensity. This Total Intensity value indicates that this odorant group, besides being the most numerous, is the strongest contributor to the overall aroma of V. pompona extract.
The development of aldehydes responsible for these fruity and fatty odors is associated with the auto-oxidation of lipids using the substrates α-linolenic and linoleic acids, which are abundant in the beans of V. pompona. The medium chain-length (C6-12) volatiles that dominate come from unsaturation of long-chain fatty acids by α- and β-oxidation, catalyzed by the enzymes galactolipase, lypoxygenase and hydroperoxide lyase during the curing process (Dunphy and Bala, 2009 and 2010).
Phenolics (vanilla, phenolic, smoky, sweet odors): Phenolic compounds such as guaiacol (see F-2), creosol and phenol (see F-3) were identified as key components of V. pompona aroma. These compounds nuanced the extract with strong, smoky, phenolic notes that also have been reported as characteristic of other commercial vanilla species (Brunschwig et al., 2012; and Perez Silva et al., 2006). Background compounds such as 4-vinyl-guaiacol, 5-vinyl-guaiacol and 4-methyl guaiacol are also contributors to the phenolic and smoky notes. The compounds p-anisaldehyde and anisyl alcohol are strong contributors to the anisic, spicy and floral aroma of the extract. The potency of the aromas from the phenolic fraction of the odorants is expressed by a sum of total intensities of 2,132, which represents an important contribution to the overall aroma of the extract.
Phenolic compounds are known to be bound as glycosides in the pods, formed during the early stages of fermentation of vanilla beans, and accumulated over time. The most studied pathway in vanilla research has been the formation of vanillin and the factors that affect its final yield. It is generally accepted that vanillin is formed by β-glucosidase-catalyzed hydrolytic cleavage of the glucoside (glucovanillin). In general, the production of the phenolic aromatic aglycones is associated with the hydrolysis of these glucosides by the Shikimic acid pathway or from interconversions of Shikimate derivatives into others (Perez Silva et al., 2006; and Dunphy and Bala, 2009).
Heterocyclics (nutty, roasted odors): Compounds that produced popcorn, roast and cheese notes such as 2-acetyl-1-pyrroline and 2-acetylpyrrole (see F-3) also highly impacted the aroma of this extract, with a sum of total intensities of 1,655.
Here, the compound ethyl pyrazine (see F-2) was not only reported in vanilla for the first time, but also was found to be a highly intensive, primary impact aroma compound, contributing with phenolic, red fruits and earthy notes to the aroma of this extract. The formation of pyrazines is associated with nonenzymatic browning and the result of microbial action.
Heterocyclic compounds are mostly products of the Maillard reaction that occurs during the process of curing—particularly during the later part of the process when chemical reactions take place in the presence of reducing sugars and sources of nitrogen, which in turn favor the formation of volatile products via the Maillard reaction. A substantial amount of work has been done to determine the pathways for the formation of individual odor compounds involved in the Maillard reaction (Reineccius, 2006; and Damodaran et al., 2008).
Carbonyl, ketones (floral, earthy odors): Several types of compounds were identified as responsible for the nuanced floral notes in the extract. However, the compound 1-5Z-octadien-3-one was attributed with the highest intensity among all the aroma-impact compounds (519). This compound produced strong floral and geranium nuances. The compound trans-geranylacetone also contributed to the floral background.
Carbonyl, ketones such as 1-octen-3-one, and 3-octen-2-one nuanced the aroma of V. pompona extract with mushroom and earthy notes. These odors were also characteristic of V. tahitensis, according to the report by Brunschwig et al. (2012). The sum of the total intensities of the odorants in this chemical fraction was 1,356.
Esters (spicy, anisic odors): Strong, spicy anise cinnamic odors come from the presence of abundant anisic compounds such as p-anisaldehyde and anisyl alcohol. However, esters such as trans-methyl cinnamate, anisyl formate and anisyl acetate were important odor contributors.
Anisic notes are not present in V. planifolia flavor and these previously have been pointed out as a fingerprint of Tahitian vanilla flavor (Brunschwig et al 2012). It is now confirmed that anisic compounds are also the backbone of V. pompona aroma. The sum of the total intensities of esters is 1,051, which represents a fair contribution.
The formation of aromatic esters has been associated with the oxidation of lipids in the presence of specific enzymes such as lypoxygenase; lipid oxidation contributes to flavor formation by the classical oxylipin pathway. Acids and ketones, among other intermediates in the oxidation process, are readily converted to alcohols, aldehydes and esters by enzymatic process during the curing process.
Aliphatic acids (cheese, sour odors): Also identified in this vanilla were short branched fatty acids, which bring cheese notes such as 3-methylbutyric acid and 2-methyl butyric acid. These also have been found to be contributors to the aromas of V. planifolia and V. tahitensis. The potency of these odors was expressed by a sum of total intensities of 904. Similar to the other two commercial vanilla species, acetic acid also was identified as a potent odorant and one of the major contributors to the aroma of V. pompona.
The formation of aliphatic acids in vanilla is associated with two alternative pathways. Branched chain acids can be biochemically generated from the corresponding Cn+1 amino acids. The route is via oxidative de-amination of the corresponding amino acid. For instance, acetic acid, a major odor contributor, can arise either by hydrolysis of acetyl-CoA formed via glycolysis or as a product of b-oxidation of even-numbered-long chain fatty acids (Dunphy and Bala, 2009).
Sulfur compounds (sulfur, earthy odors): Two compounds with sulfur odors were identified as aroma-impacting in this V. pompona extract and perceived with a sum of total intensities of 666. 2-Methyl-3-furanthiol is a primary aroma-impact compound that has previously been identified as odor-impacting in V. tahitensis by contributing to the “earthy” aroma. The compound diisopropyldisulfide is being reported for the first time in vanilla pods and it is a secondary contributor to the aroma.
According to Sarter (2011), the presence of these compounds may be due to methional development on the surface of the beans induced by thermal degradation in presence of microflora during early stages of the curing process, which should be controlled (Brunschwig et al., 2012). The amino acid methionine contains a sulfur atom that is involved in the formation of methional by the Strecker degradation reaction. The methional subsequently oxidizes to yield disulfides and thiols.
Conclusions
Wrapping up all four parts of this series, recall that first an ethanol extraction method was used to generate an aroma extract for GC-MS and GC-O analyses because it produced the most representative aroma extract of V. pompona. From the chemical characterization, 123 volatile and semi-volatile compounds were identified in V. pompona extract by GC-MS. Among the volatiles that contribute to the overall aroma quality of V. pompona extract, 45 were considered aroma-impact compounds by the GC-O method.
The aroma profile of the V. pompona extract consisted of 13 primary aroma-impact compounds, 18 secondary aroma-impact compounds and 13 odorants considered as “background.” The chemical profile of the aroma impacting compounds in V. pompona extract included 14 aldehydes, seven phenolic compounds, five heterocyclic compounds, three esters, four ketones, two acids, two alcohols, two sulfur compounds and six unknown aroma-impacting compounds.
Thus, the quality of the V. pompona aroma was found to be complex and rich with typical vanilla characteristics. Interestingly, the abundance of aroma impact compounds imprinting floral, spicy and anisic nuances to the overall typical vanilla aroma was distinctive to this species. As a result, the V. pompona species is deemed a source of aromatic compounds suitable for use in perfumery applications.
Author acknowledgements: I would like to express my appreciation to a group of experienced professionals whose valuable contributions made the accomplishment of this study possible. Thanks to the Firmenich flavorists: Bipin Kara, Usha Manduru, Ferdinand Triolo and James Stanton, for participating in the core sessions of this study. I also am deeply thankful to my advisors, Dr. Jianming Lin and Dr. Thomas G. Hartman, for their valuable guidance, assistance and encouragement throughout the course of this work.
Thank you to Dr. Jose Matiella, for his extensive assistance with the AMDIS deconvolution data analysis. Also, thanks to Ying Wang and Jackline Aoll for their assistance with the wet chemistry and instrumentation during the experimental stage of this study.
I appreciate and thank my committee members, Dr. Karen Schaich and Dr. Chi-Tang Ho, for their guidance and advice. I also am highly indebted to Dr. Scott Schneider for his willingness to help with proofreading, his scrupulous editing, and all his constructive comments.
References
J Adedeji, T Hartman and C Ho, Flavor characterization of different varieties of vanilla beans, Perfumer & Flavorist 18 115-133 (Mar 1993)
J Adedeji, Flavor characterization of different varieties of vanilla beans by direct thermal desorption-gas chromatography and gas chromatography-mass spectrometry, doctoral thesis (1993b)
C Brunschwig et al, Odor-active compounds of Tahitian vanilla flavor, Food Res Intl 46 148-157 (2012)
S Damodaran, KL Parkin and OR Fennema, Fennema’s Food Chemistry, 4th edn, CRC Press, Taylor and Francis, Boca Raton, FL USA (2008)
P Dunphy and K Bala, Vanilla curing: The senescent decline of a ripe vanilla bean and the birth of vanillin, Perfumer & Flavorist 34 34-40 (2009)
P Dunphy and K Bala, Review: A flavor of Vanilla. Aroma, taste and mouthfeel, Perfumer & Flavorist, 35 42-49 (2010)
M Galeas, Chemical Characterization of Vanilla pompona Scheide, Part I, Perfumer & Flavorist (Dec 2015)
A Perez-Silva, E Odoux, P Bart and F Ribeyre, GC-MS and GC-olfactometry analysis of aroma compounds in a representative organic aroma extract from cured Vanilla (Vanilla planifolia) beans, J Food Chem 99 728-735 (2006)
G Reineccius, Flavor Chemistry and Technology, 2nd edn, Taylor and Francis, Boca Raton, FL, USA (2006) p 242
S Sarter, in M Grisoni and E Odoux, eds, Microbial safety of cured vanilla beans, Vanilla, vol 47, CRC Press, Taylor and Francis, Boca Raton, FL USA (2011) pp 229-236