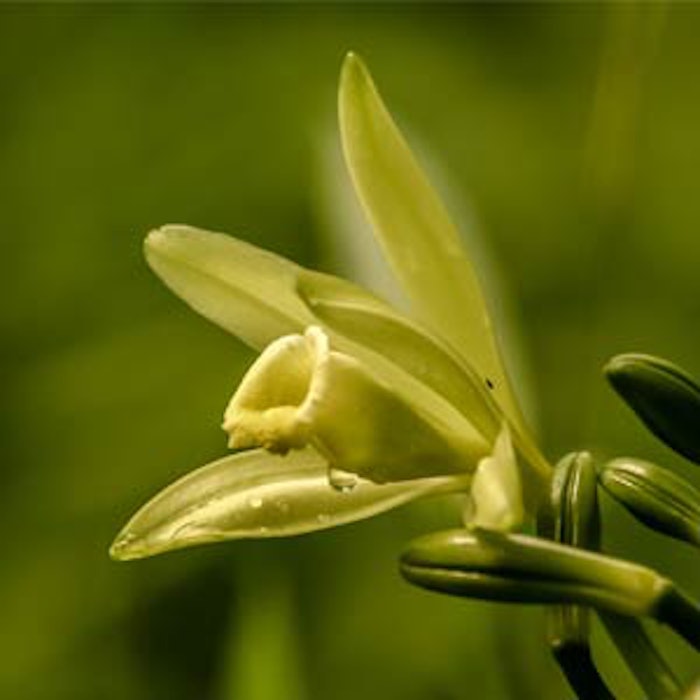
Editor’s note: This article is the third in a series of four describing the chemical characterization of Vanilla pompona. GC-MS and DTD-GC-MS were carried out in Part I to determine the ingredient’s quality as a source for aromatic compounds. Part II provided additional results and discussed overall findings. The present installment sets up the GC-O/MS protocol to further characterize the extract and provides initial observations. Part IV will detail the results and describe how the chemical distribution of V. pompona impacts its odor profile.
Published references describing the aroma quality of V. pompona are scattered. For example, Ranadive et al. (1994) reported the aroma of Guadeloupe vanilla (V. pompona) as perfumy, floral and sweet. Ehlers and Pfister (1997) reported Vanilla tahitensis and V. pompona share a strong floral/perfumy/heliotropin-like flavor, which is an attribute not found in Vanilla planifolia. Nakazawa et al. (1981) also perceived a certain similarity in the aroma quality of V. pompona to V. tahitensis, but unique anise-like notes in V. pompona.
More recently, Lee (2006) described the overall aroma quality of V. pompona beans from Madagascar as strong vanillin-like, sweet, creamy, flowery, perfumy, resinous and slightly woody with intermediate aroma characteristics between that of V. planifolia and V. tahitensis.
Given the novel aroma and attributes of V. pompona, the re-introduction of this species to international markets for use in fragrance, perfumery or pharmaceutical applications is a desirable goal of local growers, to maintain its cultivation and preserve its genetic heritage. As such, characterization of the aroma of V. pompona would validate this species as a valuable source of aromatic compounds for commercial applications. Gas chromatography-olfactometry studies were therefore carried out, as will be described.
Many volatile compounds present in vanilla are known to have odor qualities. However, the extent to which each impacts the overall odor quality can only be determined by performing gas chromatography-olfactometry (GC-O) analysis. GC-O/MS analyses of V. planifolia and V. tahitensis already have been conducted, identifying 38 aroma-impact compounds present in commercially cultivated vanilla (Perez-Silva et al. 2006; Zhang and Muller, 2012; Brunschwig et al., 2012). Additionally, 68 tentative compounds were proposed by Zhang and Mueller (2012). T-1 compiles these aroma-impact compounds, including the tentative odorants proposed by Zhang and Mueller (2012).
The complete characterization and identification of the aroma compounds in V. pompona, however, had yet to be undertaken. The present study therefore was conducted to determine the aroma-impact compounds in an extract of V. pompona Schiede by GC-O/MS analysis.
Materials
A sample of cured beans of V. pompona Schiede was obtaineda from the region of Veracruz, Mexico. The beans were grown and cured using a traditional Mexican oven and sun-drying process—green beans were scalded in an oven at 60°C for 48 hr.
Sample preparation: V. pompona extract (3X) was weighed (5 g) in a centrifuge tube using an analytical scale. Water (20 mL) and dichloromethane (DCM) (20 mL) were added to it, followed by 100 µL of chlorocyclohexane (CCH, 0.5% in methanol), measured using an analytical pipette, which served as the internal standard.
The sample was vortexed for 5 min and centrifuged for 5 min at 1,800 rpm. The upper layer phase was discarded using suction equipment and the DCM phase (bottom layer) was kept.
The sample was filtered and dried by pouring it on top of a paper filter filled with anhydrous magnesium sulfate (Mg2SO4), previously prepared. The resulting filtrate was concentrated downb to a final volume of 0.5 mL aroma extract. This aroma extract was used for GC-O/MS analysis.
GC-O/MS Data Acquisition
GC-O/MS analysis was then performed using a custom-designed systemc that integrates the gas chromatograph and mass spectrometer with an olfactory port. The eluent of the column was split between the mass spectrometer and the olfactory port at a ratio of about 1:1 via an open split interface.
Four trained GC-sniffing panelists smelled the GC effluents at the sniff port and provided their odor description using either softwarec or free vocabulary. For the former, the panelists recorded the detection times and intensity values of each perceived odor event simultaneously by pressing a button on the system panelc. The steps were repeated until completing a 50-min session, resulting in an individual aromagram. Each of the four evaluators performed the GC-sniffing session twice for a total of eight sessions, and resulting in eight single aromagrams. Each session was considered independently toward the determination of aroma-impact compounds. This number of sessions was selected according to the adequate range (6 to 12) recommended by Pollien et al. (1997).
The splitting of GC eluent between the mass spectrometer and the olfactory port allowed for the simultaneous acquisition of an individual aromagramc and a GC-MS chromatogram using GC-MS softwared. The integrated system provided the GC-O/MS information in overlay diagrams with the GC-MS chromatogram at the top and the aromagram at the bottom (see F-1). This arrangement permits a real-time comparison of the aroma characteristics provided by the panelists with the specific peaks of the chromatographic profile derived from signals measured by the MS detector.
GC-O Data Processing Approach
The approach used to process GC-O data to uncover the aroma-impact compounds in V. pompona extract could be considered a modified version of the GC-SNIF/NIF method. In this method, two parameters were used to rank the relative contributions of the aroma-active compounds perceived by a panel, to narrow them down to those compounds contributing most to the overall aroma of the extract—i.e., the aroma impact compounds. The two parameters were: the number of times each aroma event was detected, called detection frequency (DF) value; and the total of the aroma intensity (I) values assigned to each odor event. Eight GC-O runs were performed by four panelists. The olfactory data including detection times, intensity values and odor qualities collected during the GC-sniffing sessions was exported to Excel format. The data from the eight GC-O runs was aligned based on the detection times and similarity of the odor descriptions. The coincident odor events at a given detection time were combined.
The DF values were obtained by summarizing the number of recognitions and I values of each combined event from the eight individual aromagrams collected during the sessions. A composite aromagram based on the DF and total I values was thus obtained. The nasal impact frequency (NIF) value, also called “frequency,” corresponded to the percentage or number of panelists that recognized an odor event at a given retention time (Rt), with a maximum value of eight and equal to an NIF value of 100%.
The intensities of the single odor events were recorded using a scale from 1 to 100f. The sum of all I values assigned by the panelists to an odor active compound was called the Total Intensity, with a maximum value of 800. The odor events with a detection frequency of less than four and total intensity values of less than 200 were disregarded. Hence, the olfactory data was significantly reduced to only those events considered to be aroma-impact compounds.
Aroma Characteristics of the Extract
The overall aroma of the V. pompona aroma extract in this study was described by participating flavorists as vanillin, very sweet, dried fruit (pruny, raisin and fig), strong phenolic, caramel and chocolate-like, slightly floral, resinous, balsamic and heliotropin. These are considered common descriptors of commercial vanilla aromas. Thus, the overall aroma of the extract in this study was in agreement with previous descriptions, except for woody nuances, which were indicated in the preliminary study. The absence of woody notes in this extract may be attributed to differences in the origin and the curing method applied to the beans, among other factors. Both the origin of the beans and the curing process are considered key factors to define the final aroma of vanilla. In addition, experienced flavorists tend to break down single terms into several substituting descriptors. In this case, the term woody could have been substituted by resinous and balsamic.
General Remarks of the GC-O Raw Data
Vanilla is well-known for being a complex material that contains hundreds of odor active volatiles. This was evident during the GC-sniffing sessions because the complexity of the volatile profile required recording olfactory data at a very fast pace. Sniffing vanilla was particularly challenging during the most “crowded effluents section,” between 20 min and 38 min of the GC run, where the samples rapidly eluted from the capillary columns close to one another (see F-1). As expected, the individual aromagrams obtained from the GC-O sessions were similar but not exactly the same, because this is the nature of sensory data.
The bulk data from the 50-min GC sniff sessions served as building blocks for the olfactometry analysis of V. pompona extract. The raw data consisted of 523 odor events, recorded from the eight GC-sniffing sessions. Each event had a record of detection time, intensity value and aroma quality as given by the panelists. The hyphenated system provided the GC-O/MS information of each session in an overlay diagram, again with the TIC results at the top and the aromagram results at the bottom, as seen in the single run diagram shown in F-1.
In this figure, it is interesting to highlight that some potent odor compounds such as compound 4, characterized by high intensity values or peaks in the aromagram, showed little response in the GC-MS. In contrast, the most abundant chemical compounds identified by MS, such as compound 44, were not the most potent odors. Thus, the assumption that an aroma zone in the aromagram would be proportionate to a chemical compound on the TIC diagram, or vice-versa, is not always correct. In fact, most potent odorants in natural products are present at trace levels and can be difficult to detect in GC-MS data due to the presence of more abundant and less potent odor components.
Combing Aroma-active Compounds
The primary purpose of GC-O is to distinguish aroma-active compounds from volatiles without aroma. One or more aroma-active compounds are responsible for each aroma event detected during a GC-O run. Aroma events with equal odor descriptors and retention times detected in the individual GC-O runs were combined, resulting in 198 unique aroma events. The total aroma intensity and detection frequency were calculated for each. These were then plotted against their retention time to result in the composite aromagram shown in F-2.
However, it would have been difficult and time-consuming to identify each one of the 198 combined events, considering the underlying assumption of GC-O analysis is that only a small percentage of compounds are responsible for an aroma or odor of a sample (Grosch, 2001). Therefore, the first goal was to determine to what extent these volatiles contributed individually, and to select those that contributed the most to the overall aroma of V. pompona extract.
Determining Aroma-impact Compounds
The criteria for selecting aroma-impact compounds were applied to the combined odor events shown in F-2. The events having both a minimum detection frequency value of 4, i.e. 50% NIF value, and an I value over 200 were arbitrarily considered odor impact compounds. It is reasonable to assume that aroma events with higher total intensity or detection frequency contribute more significantly to the overall aroma.
The aroma events in F-2 were arbitrarily grouped into primary, secondary and background aroma-impact compounds as well as noises, based on detection frequency. The primary aroma-impact compounds were those having detection frequencies of 7 and 8, i.e., NIF values of 87.5% and 100%; the secondary aroma compounds had detection frequencies of 5 and 6, or NIF values of 62.5% and 75%; and the background aroma-impact compounds corresponded to those with detection frequencies of 4, a 50% NIF value. These categories represent the extent to which each compound contributed to the overall aroma of the vanilla extract.
Finally, the aroma events with detection frequencies of 1, 2 or 3 were considered to have no aroma impact (darkened in F-2). The removal of their “noise” from the composite aromagram led to a simplified aromagram, called the Reduced Composite Aromagram (see F-3), which contains only 45 aroma-impact peaks.
A good linear correlation (R2 = 0.8219) obtained from plotting the frequency value versus I values for each of the 45 odor events indicated the two independent criteria agreed with each other. This is in agreement with the results observed by Chaintreau (2002) for this method.
The next step in the GC-O analysis was to identify the chemical compounds responsible for the smell of these peaks. Although efforts were directed to identify the primary and secondary categories, the role of the background compounds should not be neglected since these odorants may also contribute to the balance and roundup of the overall aroma (Blank, 2002).
Identifying Aroma-impact Compounds
In general, aroma-impact compounds were identified based on their retention indices, odor qualities perceived at the sniff port, and MS; or by their retention indices, odor qualities and injection of authentic compounds. Thirty-five of the 45 aroma-impact compounds were positively identified; four were tentatively identified; and six remain unknown. These results are summarized in T-2. Since most of the unknown compounds were part of the background, it was not considered a priority to investigate their identities.
Volatile compounds responsible for those aroma events were proposed based on their similarity of odor quality and odor Retention Indices, RI(O), versus the results of RI(MS) or the retention index from libraries or publications, RI(lib). In this study, the compounds were considered acceptable when the difference was less than 20. This ID criterion is indicated as RI in T-2.
When aroma events did not match any compound from the MS data, tentative compounds were proposed based on the similarity of their RI(O) values and odor qualities with those authentic standards found in the Firmenich internal library and other databases. The compounds that comply with this ID criterion are indicated as O in T-2.
The injection of selected standard compounds to obtain the elution pattern of the compound under the instrumental conditions of this study served as confirmation in the absence of MS data or tentative compounds. This ID criterion is indicated as I in T-2. While comparing the RI(O) with the RI(I), for some of the stock odorants, the standard process was repeated several times until a suitable compound was identified. Any chemical compound that complied with at least three of the criteria was considered confirmed; otherwise, the identification was considered tentative, T.
During the identification of odorants, it was observed that identifying aroma-impact compounds based solely on MS data led, in many cases, to no identification or false identifications. This was either the result of compounds overlapping, i.e., eluting at the same time, or their intensities being below the instrument’s sensitivity. Identifying such components was therefore a challenging task. Examples were compounds #5 and #45 on the single run aromagram (see F-1). Their absence from the GC-MS data can be explained by the low odor threshold of these compounds.
The RI(O) parameter, based on the average detection time of the odor compounds, was a useful resource that, along with the odor quality, narrowed the tentative compound options.
Now that the process and the methods in each step of the process have been described and explained, the authors will present the findings of the aroma-impact compounds in found in V. pompona in the fourth and final installment of this series, to be published in March 2016.
References
I Blank, Gas chromatography-olfactometry in food aroma analysis, in R Marsili, ed, Flavor, Fragance and Odor Analysis, Marcel Dekker Inc, NY (2002) pp 297-332
C Brunschwig et al, Odor-active compounds of Tahitian vanilla flavor, Food Res Intl 46 148-157 (2012)
A Chaintreau, Quantitative use of gas chromatography-olfactometry: The GC-”SNIF” method, in R Marsili, ed, Flavor, Fragance and Odor Analysis, Marcel Dekker Inc, NY 333-348 (2002)
AAP Da Silva, DS Lundhal and MR McDaniel, The capability and psychophysics of Osme: A new GC-olfactometry technique, in H Maarse, DG van der Heih, eds, Trends in Flavour Research, Elsevier Science, Amsterdam (1994)
D Ehlers and M Pfister, Compounds of vanillons (Vanilla pompona Schiede), J Essential Oil Res 9 427-431 (1997)
W Grosch, Evaluation of the key odorants of foods by dilution experiments, aroma models and omission, Chem Senses 28 533-545 (2001)
KJ Lee, Analytical characterization of volatile and semi-volatile constituents of vanilla beans produced in different geographical regions, Rutgers University, master’s thesis (2006)
Y Nakazawa et al, Studies on the vanilla flavors for food processing III. Characteristics of natural vanilla flavors from several beans produced in the some countries of the world, Japanese J Dairy and Food Science 30 116-123 (1981)
A Perez-Silva, E Odoux, P Bart and F Ribeyre, GC-MS and GC-olfactometry analysis of aroma compounds in a representative organic aroma extract from cured Vanilla (Vanilla planifolia) beans, J Food Chemistry 99 728-735 (2006)
P Pollien, A Ott, F Montigon, M Baumgartner, R Munoz-Box and A Chaintreau, Hyphenated headspace-gas chromatography-sniffing technique: Screening of impact odorants and quantitative aromagram comparisons, J Agricultural and Food Chem 45 2630-2637 (1997)
AS Ranadive, Vanilla-cultivation, curing, chemistry, technology and commercial product, in G Charalambous, ed, Spices Herbs and Edible Fungi. Dev in Food Science, vol. 34, Elsevier, Amsterdam (1994) pp 517-577
S Zhang and C Mueller, Comparative analysis of volatiles in traditionally cured Bourbon and Ugandan vanilla bean (Vanilla planifolia) extracts, J Agricultural and Food Chem 60 10433−10444 (2012)